Spectral/hp element methods for turbomachinery
CFD is now an indispensable tool for the design of aircraft engines, and it has become commonplace in the design guidance of new technologies and products. In order for CFD to be effectively adopted in industry, validation and verification is required over a broad design space. This is challenging for a number of reasons, including the range of operating conditions (i.e. Reynolds numbers, Mach numbers, temperatures and pressures), the complexity of industrial geometries (including uncertainty due to manufacturing variations) and their relative motion (i.e. rotor-stator interactions). Even though RANS continues to be the backbone of CFD-based design, the recent development of high-order unstructured solvers and high-order unstructured meshing algorithms, combined with the lowering cost of HPC infrastructures, has the potential to allow for the introduction of high-fidelity transient simulations using large-eddy or direct numerical simulations (LES/DNS) in the design loop, taking the role of a virtual wind tunnel.
The first part of this Rolls-Royce funded project focused on the numerical properties of the spectral/hp element methods applied to turbomachinery: A T106A low pressure turbine vane was investigated at moderate regime (Re = 88450) using Nektar++, exploring the convergence properties of the main flow statistics with the aim of developing a set of best practices to achieve DNS resolution [1]. In this study we quantified the computational cost required for given degree of accuracy in performance prediction, and the results showed excellent agreement with experimental findings.
In this test case, transition to turbulence takes place only in the final portion of the suction surface, where the separated shear layer rolls up due to Kevin-Helmoltz instability (visually represented in the figure below). The separation bubble on the suction surface remains open and merges into the trailing edge wake, giving rise to large-scale vortical structures.
This work was conducted with clean inflow conditions to isolate the effect of the numerical setup on the various flow statistics.
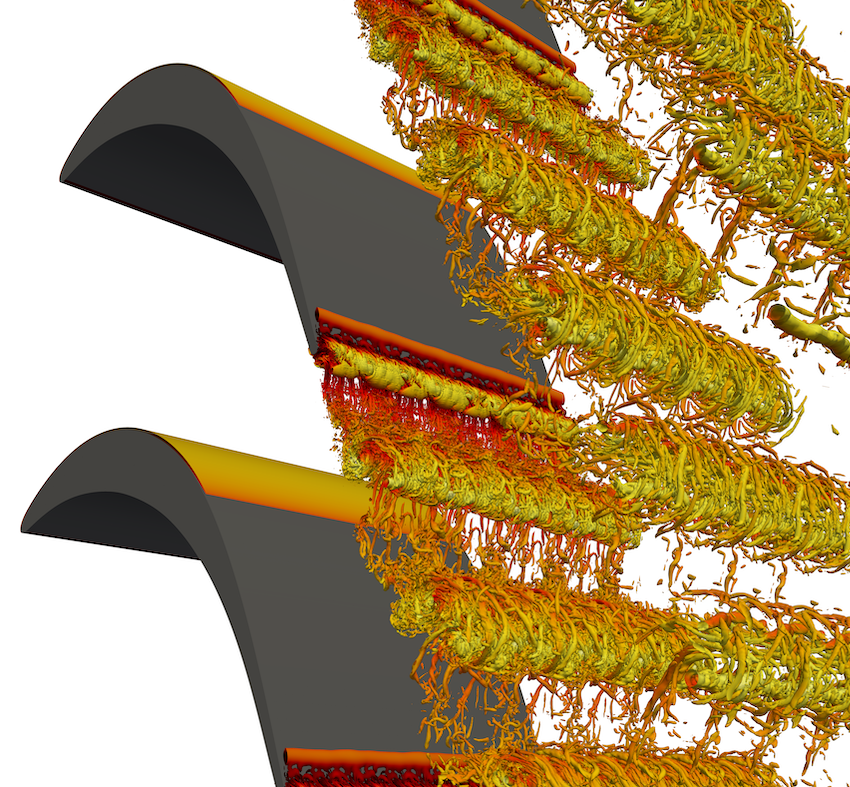
Figure 1: Instantaneous isosurfaces of Q-criterion (Q = 500) contoured by velocity magnitude, showing the vortical structures evolving on the suction surface and in the wake of a T106A cascade.
However, turbomachinery flows are extremely complex and highly turbulent, with both deterministic and stochastic contributions. The former arises from stator-rotor interaction, while the latter is due to turbulence. Their interaction is yet to be fully understood, and it crucially controls the majority of irreversibilities.
We have subsequently focused on the analysis of the impact of stochastic noise on the main cascade statistics, implementing two methodologies for synthetically generating turbulence on the fly. Their effect was explored for the first time on a low pressure cascade, to gain understanding on how to best simulate a realistic environment [2].
The gallery below shows the kind of turbulent structures generated using these two approaches, based on localised momentum forcing (on the left) and synthetic inflow turbulence generation (on the right).
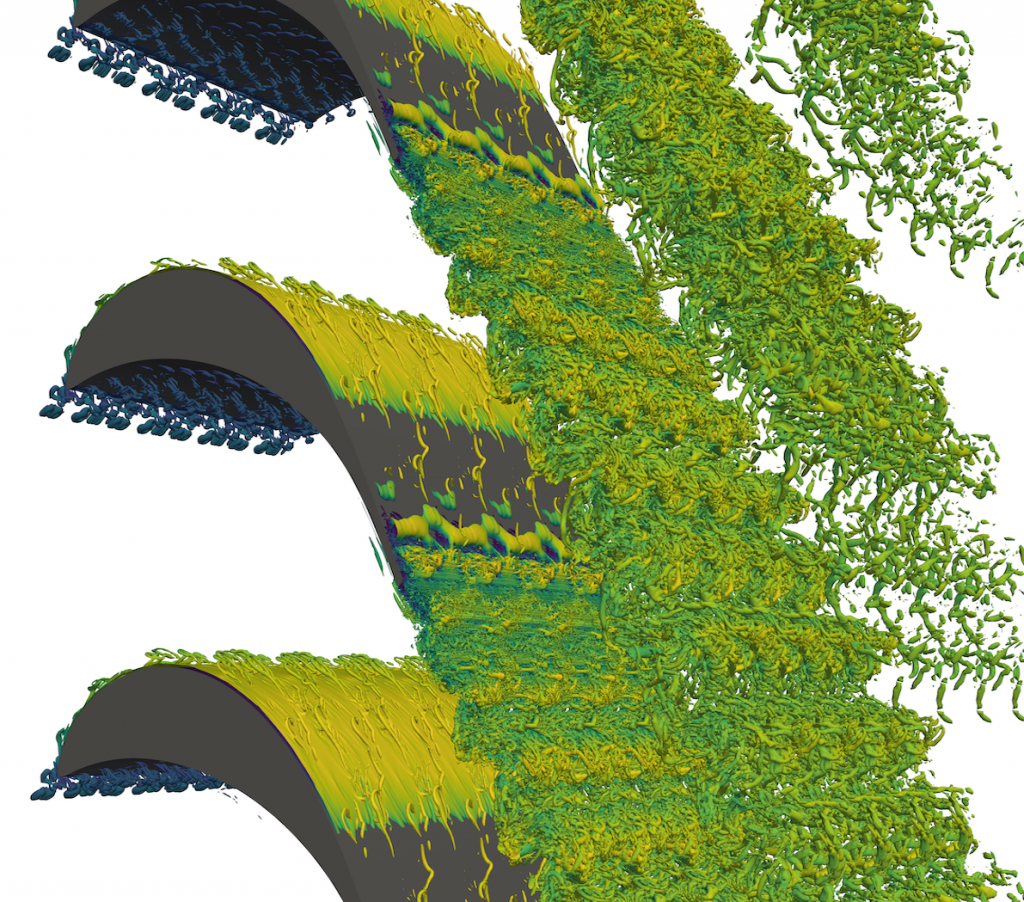
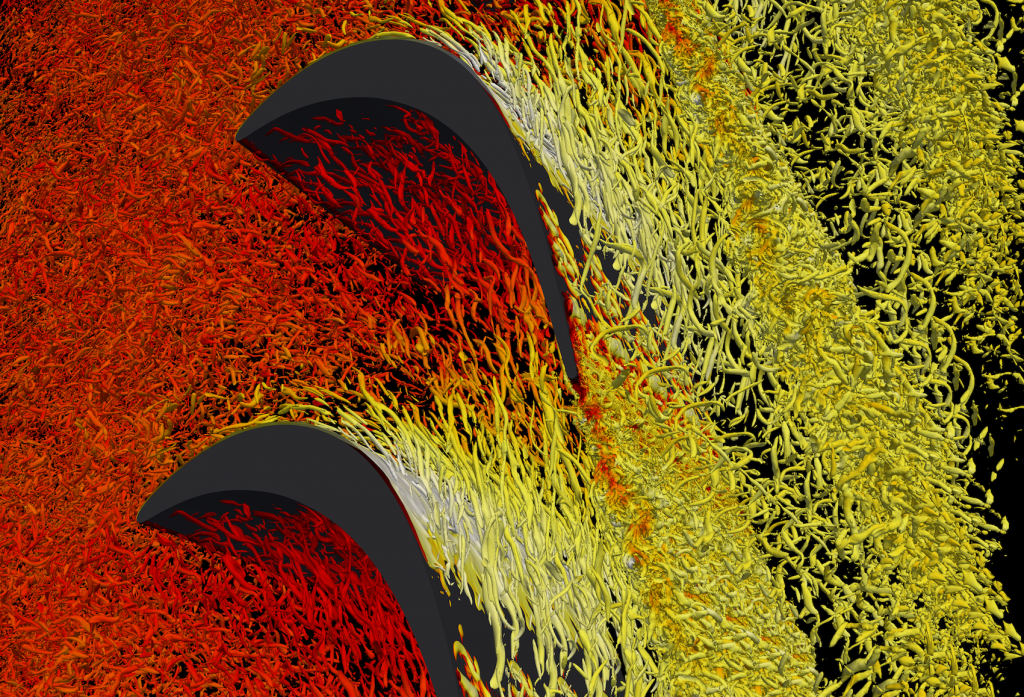